Season 2 of Orphan Black left us with a couple of doozies. First and foremost, we found there is another group of clones, created as part of Project Leda’s militaristic male clone companion project, Project Castor. And so far those clones have been wreaking all sorts of havoc in season 3. But we also found out that the scholarly clone Orphan Black Wikia - Cosima Niehaus (Tatiana Maslany) is perhaps dying . Luckily, Cosima is a biologist currently working from inside the maligned Dyad Institute, which is now run by her love interest, Orphan Black Wikia - Delphine Cormier (Évelyne Brochu), and the stem cell treatment derived from a baby tooth lost by Orphan Black Wikia - Kira Manning (Skyler Wexler) appears to be working (as of episode 2 of season 3) so at least Cosima’s fate rests in her own hands. While Cosima isn’t a medical doctor or a human reproductive biologist, she still has a highly applicable knowledge base. Every single time a new science bombshell is dropped on the Orphan Black viewing audience, it is always either coming from or being explained by Cosima.
How does Cosima have all the answers?
As mentioned throughout season 1 of Orphan Black, probably a dozen or more times, Cosima studies evolutionary developmental biology, which is often shortened to the far catchier evo-devo. So what is evo-devo? Is it even a real field of research? And if so, what has it told us about the living world? To answer those questions, we should first delve into a little bit of history of biology.
A very brief history of developmental biology

Most people are at least vaguely familiar with the study of evolution, kick started by two Victorian Englishmen, the famous Charles Darwin and the sadly overlooked Alfred Russel Wallace, co-discoverers of natural selection. However, before either fellow suggested a single common origin of all life on earth, people had been studying embryogenesis or development, the process by which a single-celled zygote becomes a full-fledged organism. For example, German-speaking Estonian Karl Ernst von Baer had pointed out some jarring features shared by developing mammal, bird, reptile, and even fish embryos. Upon examining the embryos and fetuses of every animal he could get his hands on, von Baer found that all vertebrate animals started off as very similar looking embryos and that specific types of organisms, such as mammals, birds, or fishes, developed similarly for longer. Put another way, von Baer found that 2 weeks after fertilization, all animal embryos were indistinguishable. But after 2 months, developing mammals still resembled each other and developing birds still resembled each other, but these mammals and birds fetuses were quite distinct from each other. A couple months later, the fetuses of mammals like pigs and humans no longer resembled each other.
After the outrageously successful publication of the The Origin of Species, scientists (then called naturalists or natural philosophers) started recasting previously discovered biological phenomena in terms of evolution. As such, folks like German-speaking Prussian Ernst Haeckel reinterpreted von Baer’s observation in light of evolution, claiming that development itself evolves, giving rise to evolved adult forms.
What von Baer and Haeckel had stumbled upon was the concept of homology, or shared structures or characteristics of different types of organisms that can develop in divergent ways. (And may or may not be retained into adulthood. For example, mice, fish, frogs and humans embryos all share the homology of an embryonic tail, but by the end of frog and human development, both species lack tails.)
Put another way, appendages such as bird wings and human arms originate from the same, homologous structures, but over time the structures that form wings and arms begin to diverge. If you watch human hands and chicken wings develop, they are very similar at first, but become distinct over time. Wing tips are stretched out hands with webbed skin, while human hands are small wing tips without all that webbing. This was a neat observation, and it was limited to being a merely neat observation until we developed the tools to truly appreciate its implications.
More history, even more quickly

Fast forward 40 years to 1900, and the pioneering work of a German-speaking Czech monk, Gregor Mendel, (where the heck are all these Germans coming from?) is rediscovered, and shortly all biologists know what genes are. Fast forward another 50 years, and the Hershey–Chase experiment proves DNA is what genes are made out of. Fast forward just another couple decades to the late 1970s, and scientists then began to develop the molecular genetic tools that allow for detection and manipulation of the actual DNA, RNA, and proteins that are produced by each gene. By the early 1980s, a series of techniques began to coalesce. Methods like antibody-based staining, molecular cloning, in situ hybridization, and eventually polymerase chain reactions, RNA interference, quantitative PCR, and microarray analysis were applied to model organisms ranging from humans, mice, and chickens to zebra fish, fruit flies, and sea urchins.
These methods are rather technical so I won’t bore you with their details, but suffice it to say, they permit the direct observation of genes within developing organisms. Initially, these techniques were used within species to determine the genes associated with the development of structures, giving rise to the field of developmental genetics in the late 1970s. Discoveries like the Hox genes in fruit flies in 1978 (for which Cal Tech geneticist Edward Lewis later won a 1995 Nobel Prize) began to show how organisms were constructed. In other words, the mystery behind how a gene produces visible traits was beginning to be revealed.
Eventually, enough of these studies were being done, on both closely and distantly related species, that by the late 1980s, scientists could suddenly compare the development of vastly different organisms that had evolved into very distinct species. In other words, the field of evolutionary developmental biology, or evo-devo, had emerged.
What has evo-devo shown us?
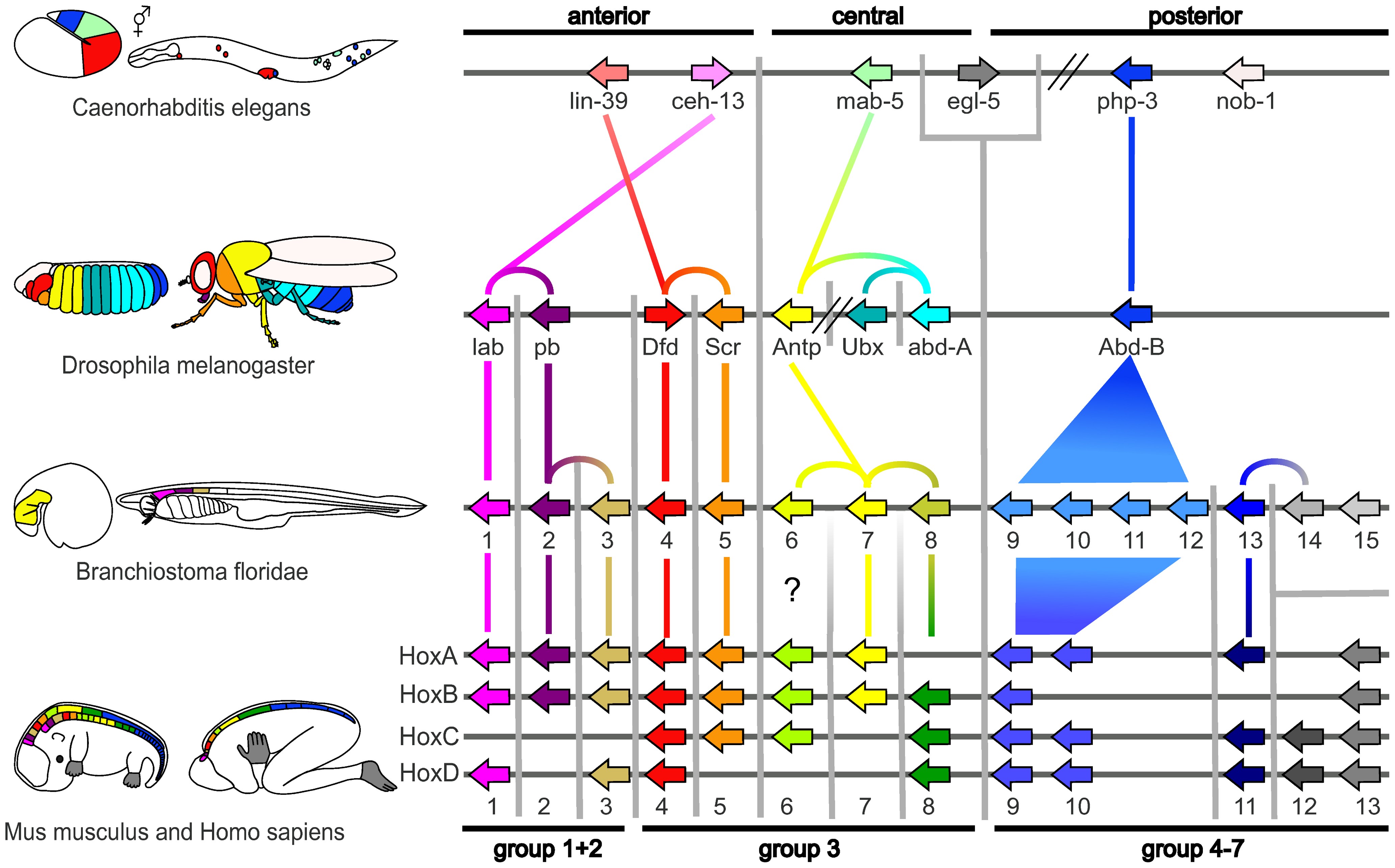
Back when genomics was just a pipe dream—when it seemed impossible to sequence a genome (never mind author a genome, as reviewed in an earlier blog post)—the field of evo-devo was blazing hot, illuminating some of the longstanding mysteries of life. The overarching goal of evo-devo is to understand how the link between genes and characteristics of organisms evolves. Effectively, evo-devo seeks to understand why we won’t look like fruit flies, even though we share thousands of genes with them.
That the same genes can produce homologous (but divergent) structures in wildly different organisms is one of the major results of evo-devo. For example, the same cluster of Hox genes, found in the same order within vastly different genomes, are responsible for establishing the overall body structure of both fruit flies and mice, despite the fact that their ancestors diverged over half a billion years ago. Somehow, over this time, evolution has transformed the organisms that are presently mice and fruit flies, but maintained the genes that produce these different morphologies. Furthermore, Hox genes are so well conserved that you can break a fruit fly Hox gene with mutations and then replace it with a chicken Hox gene and still get a viable fruit fly (extra credit scientific article here).
While this might sound like a minor finding, consider this. Every time researchers want to understand a human disease or consider the basic principal for a new drug, it is incredibly difficult, and often unethical to perform the appropriate studies on humans. By understanding the similarities in development and disease in humans and other species, organisms as divergent as pigs and (again) fruit flies, can be used as model systems to determine what is happening in humans.
How has this helped Cosima understand what’s happening to her and the other clones?
In brief, Cosima has all the insight because before she was swept away from her PhD program at the University of Minnesota, she was working towards her degree in evo-devo, an incredibly integrative field that requires an expansive knowledge and skill base. Evo-devo concentrates on determining how organisms are built—how genomes full of genes become plants and animals of all shapes and sizes that fill our world—and modern evo-devo does so using all the technology and methodologies developed by 19th century embryologists, 20th century evolutionary biologists, and 21st century genomicists. If anyone in the Orphan Black universe can figure out how the synthetic sequences of the clones are mucking up their reproductive capacity and health, it’s Cosima.
Once again the writers of Orphan Black have maintained a remarkable degree of scientific realism in their world. Not only does the science of the show jive with reality, but people like Orphan Black science consultant Cosima Herter (is that name a coincidence?) even made sure that one of the clones had the background to legitimately explain what the heck is going on with these clones. We live in a remarkable world, where the things we do and see every single day were beyond the faintest imagination of